What is Root Cause Analysis (RCA)?
Definition and Purpose of RCA
Root Cause Analysis (RCA) is a structured problem-solving methodology aimed at identifying the underlying causes of an issue rather than addressing its surface symptoms. By uncovering and addressing the root cause, organizations can implement solutions that prevent the recurrence of the problem, leading to long-term operational improvements.
The purpose of RCA is to:
- Systematically investigate problems to identify their fundamental causes.
- Design corrective actions that eliminate or mitigate these causes.
- Improve reliability, efficiency, and safety within systems and processes.
In manufacturing and industrial automation, RCA is essential because the complexity of systems often leads to intertwined issues. Addressing symptoms without understanding root causes can lead to recurring failures, unplanned downtime, and increased operational costs.
Importance of RCA in Manufacturing and Industrial Automation
In the high-stakes world of manufacturing, downtime, quality defects, and safety incidents can result in significant financial and reputational losses. RCA provides a disciplined approach to problem-solving, offering several critical advantages:
- Minimizing Downtime: RCA identifies and resolves issues that lead to machine or process failures, ensuring uninterrupted production.
- Enhancing Quality: By addressing the root causes of defects, manufacturers can achieve consistent product quality, reducing waste and rework.
- Improving Safety: RCA helps uncover hazards and implement controls to prevent accidents, fostering a safer workplace.
- Optimizing Costs: Avoiding recurring problems leads to cost savings by reducing emergency repairs, scrap, and inefficiencies.
- Supporting Predictive Maintenance: RCA integrates well with Industry 4.0 technologies like IoT and predictive analytics, helping to preempt failures before they occur.
For industrial automation systems, RCA is particularly vital as these systems involve complex interactions between hardware, software, and human operators. RCA ensures that automation systems deliver consistent performance and reliability.
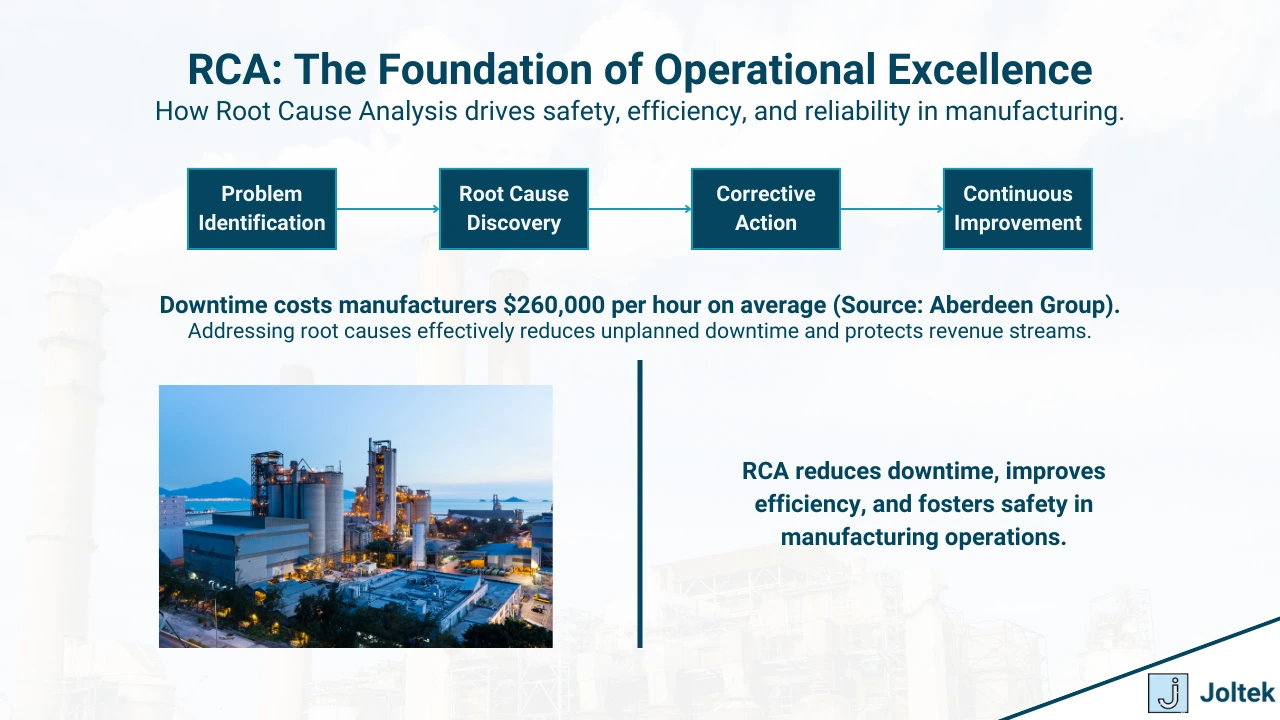
Common Myths and Misconceptions About RCA
- “RCA Always Identifies All Causes of a Problem”
- Reality: While RCA aims to uncover root causes, some issues are influenced by complex or poorly understood interactions, making it challenging to identify all contributing factors. The goal is to find actionable causes that significantly reduce the likelihood of recurrence.
- “RCA is Only Necessary for Big Problems”
- Reality: RCA is equally effective for smaller, recurring issues. Small problems left unaddressed can escalate into significant failures over time.
- “RCA is a One-Time Activity”
- Reality: RCA is iterative and continuous. Organizations should revisit RCA findings as systems evolve and new data becomes available.
- “RCA is Time-Consuming and Expensive”
- Reality: While RCA requires an upfront investment of time and resources, it saves costs in the long term by preventing problem recurrence.
Benefits of Implementing RCA in Manufacturing
- Improved Efficiency
- Resolving bottlenecks and inefficiencies through RCA leads to streamlined processes, higher throughput, and better resource utilization.
- Example: A bottling plant identified irregular filling speeds as the root cause of production delays. RCA led to adjustments in machine calibration, improving production by 15%.
- Reduced Downtime
- RCA minimizes downtime by addressing chronic equipment failures. Proactive RCA can also be integrated with predictive maintenance strategies.
- Example: Identifying overheating as a root cause for motor failures saved a manufacturer $100,000 annually in downtime costs.
- Cost Savings
- RCA reduces costs associated with waste, defects, and emergency repairs. It also prevents costly safety incidents and liability claims.
- Example: A food processing facility identified the root cause of packaging defects, reducing scrap material by 25% and saving $50,000 annually.
- Cultural Shift Toward Problem-Solving
- RCA promotes a culture of continuous improvement, encouraging teams to approach problems systematically and collaboratively.
Context of the Article: Consulting, Training, and Systems Integration
At its core, RCA aligns seamlessly with consulting, training, and systems integration services in manufacturing and industrial automation:
- Consulting: Helping organizations design and implement RCA frameworks tailored to their operations.
- Training: Equipping teams with the skills to conduct effective RCA, use tools like Fishbone Diagrams and 5 Whys, and foster a culture of problem-solving.
- Systems Integration: Leveraging RCA to identify system inefficiencies and implement integrated solutions, such as automation upgrades, MES/ERP integrations, and IoT-based monitoring systems.
By embedding RCA into consulting and integration practices, organizations can achieve sustainable improvements and competitive advantages in their manufacturing operations.
The Importance of Root Cause Analysis in Manufacturing
Why RCA is Critical in High-Stakes Industries Like Manufacturing
In high-stakes industries such as manufacturing, where production efficiency and system reliability are paramount, the ability to identify and eliminate underlying problems is essential. Root Cause Analysis (RCA) provides a structured approach to uncovering the fundamental causes of issues rather than simply addressing their symptoms. This distinction is critical because surface-level fixes often lead to recurring problems, resulting in wasted resources, production delays, and diminished profitability.
For example, in a facility producing automotive components, repeated downtime in a key assembly line was initially blamed on operator error. However, an RCA investigation revealed that miscalibrated sensors were intermittently stopping the line. Addressing this root cause not only eliminated the downtime but also improved operator efficiency and morale. RCA enables manufacturers to tackle such systemic issues, yielding benefits like minimized downtime, improved product quality, and safer workplaces.
Without RCA, high-stakes environments are vulnerable to what can be described as “problem snowballing.” An unresolved minor issue, like inconsistent machine performance, can cascade into larger failures that disrupt entire operations. In industries where a single hour of downtime can cost hundreds of thousands of dollars, this ripple effect can be catastrophic. RCA stands as a preventive shield, ensuring small problems are stopped in their tracks before they become large-scale crises.
Challenges Faced Without Effective RCA
When RCA is overlooked or improperly executed, manufacturing facilities often find themselves trapped in cycles of inefficiency and recurring failures. One major issue is the recurrence of equipment failures. Many organizations, for example, replace worn components without investigating why the components wore out prematurely. This approach leads to repeated failures, increased repair costs, and avoidable downtime. RCA helps break this cycle by delving into the deeper reasons behind component wear, such as misalignment, environmental factors, or suboptimal operating conditions.
Similarly, production delays are another common consequence of failing to perform proper RCA. In many cases, delays are mistakenly attributed to isolated issues, like machine operators not meeting quotas or machines performing below specifications. However, RCA often reveals interconnected causes. For instance, in a bottling plant experiencing frequent slowdowns, RCA identified irregularities in sensor feedback as the root cause, which were then corrected to streamline operations.
High maintenance costs are another key challenge linked to a lack of RCA. Reactive maintenance—responding to problems after they arise—is both expensive and inefficient. Without RCA, maintenance teams may address symptoms like repeated bearing failures without uncovering the root cause, such as excessive vibration or improper lubrication. By implementing RCA, organizations can transition to predictive or preventive maintenance strategies, reducing costs and improving equipment reliability.
RCA’s Role in Digital Transformation and Industry 4.0
The integration of digital technologies into manufacturing processes, often referred to as Industry 4.0, has amplified the importance of RCA. Advanced technologies like IoT sensors, machine learning algorithms, and real-time monitoring systems provide unprecedented access to data. RCA leverages this data to pinpoint and address root causes more effectively and efficiently.
For example, predictive maintenance tools powered by IoT sensors can alert teams to anomalies, such as excessive vibration in a critical pump. However, while these tools identify symptoms, RCA ensures that the underlying causes are addressed. In this case, RCA might uncover that the pump was incorrectly installed or that material quality issues are causing stress on components. By combining digital tools with RCA, manufacturers can move beyond symptom detection to achieve true problem resolution.
Digital transformation also accelerates problem-solving through tools like digital twins, which create virtual models of manufacturing systems. These models allow teams to simulate potential failure scenarios and identify root causes in a virtual environment, reducing downtime during the investigation process. Moreover, centralized data systems enable cross-functional teams to collaborate on RCA, sharing insights and findings across departments to drive faster, more holistic solutions.
Why RCA is a Cornerstone of Excellence in Manufacturing
The benefits of implementing RCA in manufacturing extend far beyond problem-solving. RCA fosters a culture of continuous improvement by encouraging teams to ask not only “what went wrong?” but also “how can we ensure it doesn’t happen again?” This mindset aligns perfectly with lean manufacturing principles, which prioritize waste elimination and efficiency optimization.
Moreover, RCA contributes to significant cost savings. In one case study, a food packaging facility was experiencing high levels of scrap material due to misaligned sealing equipment. Through RCA, the team identified inconsistencies in operator training as the root cause. By addressing this training gap, the facility reduced scrap by 30%, saving over $80,000 annually.
In the context of consulting, training, and systems integration, RCA provides a tangible framework for delivering measurable results. Whether it’s conducting a thorough investigation into recurring equipment failures, training teams to implement effective RCA techniques, or integrating RCA with predictive analytics systems, the approach ensures long-term operational gains. In manufacturing, where every decision impacts the bottom line, RCA is not just a tool—it is a competitive advantage.
Overview of 7 Root Cause Analysis Techniques
Root Cause Analysis (RCA) is a versatile framework that can be adapted to various challenges in manufacturing and industrial automation. Different situations call for different tools, each with its unique strengths and applications. Below is an overview of seven commonly used RCA techniques, their principles, and how they contribute to effective problem-solving.

1. Ishikawa Fishbone Diagram (IFD)
Also known as the Cause-and-Effect or Fishbone Diagram, this technique is ideal for identifying and categorizing potential causes of a problem. Developed by Kaoru Ishikawa, it visually organizes contributing factors into categories like People, Process, Equipment, and Materials, making it easier to uncover relationships between causes and effects.
- When to Use: When you need a structured brainstorming tool to explore the root causes of a recurring issue.
- Example: Diagnosing downtime in a packaging line by analyzing equipment failures, operator actions, and environmental conditions.
Key takeaway: The Fishbone Diagram’s structured approach makes it especially useful for tackling complex, multifactorial issues.
2. Pareto Chart
The Pareto Chart applies the 80/20 rule, focusing on the "vital few" causes that contribute to the majority of a problem. It uses a combination of bar and line graphs to prioritize issues based on their frequency or impact, allowing teams to concentrate their efforts where they’ll have the most significant results.
- When to Use: When you need to prioritize problems to address the most critical ones first.
- Example: Identifying that 80% of defects in a production line come from just 20% of machine setups.
Key takeaway: The Pareto Chart is an excellent tool for data-driven decision-making and resource allocation in RCA.
3. 5 Whys
The 5 Whys technique is a simple yet powerful method for drilling down into the root cause of a problem by repeatedly asking "why" until the fundamental issue is revealed. Its simplicity and accessibility make it one of the most widely used RCA tools.
- When to Use: For straightforward problems requiring quick insights without extensive data analysis.
- Example: Investigating why a conveyor belt stopped mid-production and discovering an overlooked lubrication schedule as the root cause.
Key takeaway: The 5 Whys is highly effective when applied rigorously, but it requires discipline to avoid subjective or superficial answers.
4. Failure Mode and Effects Analysis (FMEA)
FMEA is a proactive technique that identifies potential failure modes, their causes, and their impacts before they occur. It evaluates each failure mode based on severity, occurrence, and detection likelihood, assigning a Risk Priority Number (RPN) to prioritize corrective actions.
- When to Use: During system design or maintenance planning to predict and mitigate risks.
- Example: Anticipating potential causes of robotic arm malfunctions in a new assembly line and addressing them before deployment.
Key takeaway: FMEA’s quantitative approach makes it indispensable for risk management and preventive maintenance strategies.
5. Fault Tree Analysis (FTA)
FTA is a deductive tool that maps out potential causes of a problem in a tree-like structure, starting with the undesired event at the top and branching downward into contributing factors. It incorporates probabilities to assess the likelihood of different failure paths.
- When to Use: For complex systems where multiple factors could lead to a failure.
- Example: Determining the root causes of intermittent electrical faults in an automated process.
Key takeaway: FTA provides a logical, visual representation of potential failure scenarios, making it valuable for high-complexity systems.
6. Affinity Diagram
The Affinity Diagram is a collaborative tool that organizes large sets of ideas or data into meaningful categories. It is particularly useful in group settings to identify patterns and relationships that may not be immediately obvious.
- When to Use: When dealing with ambiguous or loosely defined problems requiring team input.
- Example: Grouping feedback from operators to identify recurring themes behind downtime incidents.
Key takeaway: The Affinity Diagram fosters team collaboration and helps uncover insights through categorization.
7. Scatter Diagram
A Scatter Diagram, or Scatter Plot, is a statistical tool that plots two variables to determine their relationship. By analyzing the correlation between variables, it helps identify possible root causes based on data patterns.
- When to Use: When you need to test hypotheses about relationships between variables.
- Example: Exploring the link between machine vibration levels and production defects.
Key takeaway: Scatter Diagrams excel at uncovering data-driven relationships, making them indispensable in scenarios where precision is key.
Conclusion
These seven RCA techniques each serve a unique purpose in manufacturing and industrial automation. From brainstorming and prioritization to risk assessment and statistical analysis, they offer diverse approaches to identifying and resolving root causes. By mastering and applying these techniques appropriately, manufacturers can achieve significant gains in efficiency, quality, and reliability. Whether used individually or in combination, these tools are essential components of a robust RCA framework.
Ishikawa Fishbone Diagram (IFD): A Detailed Breakdown
History and Development by Kaoru Ishikawa
The Ishikawa Fishbone Diagram, also known as the Cause-and-Effect Diagram, was developed by Kaoru Ishikawa in the 1960s. Ishikawa, a pioneer in quality management, created this tool to help teams systematically identify and address the root causes of problems. Its name comes from its resemblance to a fish skeleton, with the “head” representing the problem and the “bones” representing potential causes. Ishikawa introduced this diagram as part of his broader contributions to quality control, which also included the promotion of Total Quality Management (TQM) principles in Japan.
The Fishbone Diagram became widely adopted across industries due to its simplicity and effectiveness, particularly in manufacturing environments. It remains a foundational tool in problem-solving, quality assurance, and lean manufacturing initiatives.
How to Create and Use a Fishbone Diagram
Creating a Fishbone Diagram involves a collaborative, structured approach to problem-solving. The steps are as follows:
- Define the Problem: Clearly articulate the issue you want to analyze. Place it at the “head” of the diagram.
- Example: “Equipment malfunctions in a packaging line.”
- Identify Major Categories: Determine broad categories that might contribute to the problem. Common categories in manufacturing include:
- People: Operator skills, training, communication.
- Process: Procedures, workflows, standardization.
- Equipment: Machinery, tools, maintenance.
- Materials: Quality, availability, specifications.
- Environment: Workspace conditions, temperature, humidity.
- Brainstorm Potential Causes: Under each category, brainstorm specific potential causes. Draw “bones” branching from each category to list these causes.
- Equipment: Worn bearings, misaligned sensors, lack of lubrication.
- People: Insufficient training, operator fatigue, unclear instructions.
- Analyze and Prioritize Causes: Review the potential causes and identify those most likely contributing to the problem. Use data, observations, or additional analysis to validate your findings.
- Develop Solutions: Based on the identified root causes, propose actionable solutions to eliminate or mitigate the issue. Implement these solutions and monitor their effectiveness.
Example: Analyzing Equipment Malfunctions in a Packaging Line
Scenario: A packaging line in a manufacturing facility is experiencing frequent downtime, leading to missed production targets. An RCA team is tasked with identifying the root causes.
- Define the Problem:
- Problem Statement: The packaging line experiences equipment malfunctions twice daily, reducing productivity by 20%.
- Identify Major Categories:
- People, Process, Equipment, Materials, Environment.
- Brainstorm Potential Causes:
- People: Operators unfamiliar with troubleshooting procedures.
- Process: Irregular maintenance schedules.
- Equipment: Worn conveyor belts, misaligned labeling machines.
- Materials: Packaging film inconsistencies causing jams.
- Environment: Dust buildup affecting sensors.
- Analyze and Prioritize Causes
- Historical maintenance logs reveal that conveyor belt issues account for 70% of downtime. Additional inspection confirms that poor alignment of the labeling machine exacerbates the problem.
- Develop Solutions:
- Solution 1: Replace worn conveyor belts and introduce predictive maintenance using IoT sensors.
- Solution 2: Realign and recalibrate labeling machines, followed by operator retraining.
- Solution 3: Enhance packaging material quality control checks.
- Implementation and Monitoring:
- Solutions are implemented over three weeks, and IoT sensors provide real-time feedback. Downtime decreases by 90%, saving the facility $25,000 per month.
Why the Fishbone Diagram is Effective
The Ishikawa Fishbone Diagram excels in its ability to organize complex problems into digestible components. It encourages collaboration, promotes critical thinking, and provides a visual representation of cause-and-effect relationships. This makes it especially valuable in manufacturing environments, where multiple factors often interplay in causing issues. When used alongside data-driven tools like Pareto Charts or IoT monitoring systems, the Fishbone Diagram becomes even more powerful in driving actionable solutions.
Pareto Chart: A Detailed Breakdown
The 80/20 Rule and Its Application in Manufacturing
The Pareto Chart is based on the 80/20 rule, or the Pareto Principle, which states that 80% of outcomes are often driven by 20% of causes. Named after Italian economist Vilfredo Pareto, who observed that 80% of Italy’s land was owned by 20% of the population, this principle has been widely applied to problem-solving and prioritization in various industries, including manufacturing.
In manufacturing, the 80/20 rule suggests that a small number of factors often account for the majority of problems, defects, or inefficiencies. For instance, 80% of production downtime might be caused by 20% of equipment issues, or 80% of quality defects might stem from 20% of process variations. The Pareto Chart visually represents this relationship, helping teams focus their efforts on the few high-impact causes rather than spreading resources thinly across numerous minor issues.

How Pareto Charts Help Prioritize Problems
A Pareto Chart is a combination of a bar graph and a line graph. The bars represent the frequency or impact of individual causes, arranged in descending order, while the line shows the cumulative percentage of the total impact. This visual format makes it easy to identify the "vital few" causes that contribute the most to a problem.
Steps to Create and Use a Pareto Chart:
- Collect Data: Gather data on the issue you want to analyze, such as the number of defects, occurrences of downtime, or financial losses related to specific problems.
- Categorize Causes: Group the data into categories (e.g., machine types, defect types, process steps).
- Sort and Rank: Arrange the categories by their frequency or impact in descending order.
- Calculate Cumulative Percentages: Determine the cumulative percentage for each category.
- Visualize the Data: Plot the categories as bars and overlay the cumulative percentage as a line graph.
- Identify the Vital Few: Use the 80/20 rule to identify the top contributors to the problem. These are the areas where efforts should be concentrated.
The result is a clear, actionable visualization that helps prioritize the most significant causes, allowing teams to allocate resources effectively and achieve maximum impact with minimal effort.
Example: Identifying Defects in Production Batches
Scenario: A beverage manufacturer faces a recurring issue with product defects, leading to customer complaints and increased waste. The quality assurance team decides to use a Pareto Chart to identify the primary causes of defects.
- Data Collection: Over a one-month period, the team tracks the types of defects in production batches and their frequencies:
- Label misalignment: 40 occurrences
- Bottle sealing failure: 30 occurrences
- Filling inaccuracies: 20 occurrences
- Bottle cracks: 10 occurrences
- Categorization and Sorting: The defects are grouped into categories and arranged in descending order:
- Label misalignment: 40
- Bottle sealing failure: 30
- Filling inaccuracies: 20
- Bottle cracks: 10
- Cumulative Percentages:
- Label misalignment: 40/100 = 40%
- Bottle sealing failure: 30/100 = 30% (cumulative = 70%)
- Filling inaccuracies: 20/100 = 20% (cumulative = 90%)
- Bottle cracks: 10/100 = 10% (cumulative = 100%)
- Visualization:
- A Pareto Chart is created with the categories as bars representing defect frequencies and a line graph showing cumulative percentages.
- Insights and Action:
- The chart reveals that label misalignment and bottle sealing failures account for 70% of all defects. The team focuses on these two areas, discovering that label misalignment is caused by a misaligned labeling machine and sealing failures result from worn gaskets on the capping machine. Corrective actions include recalibrating the labeling equipment and replacing the gaskets.
Results: After addressing the top two defect categories, overall defects decrease by 65%, saving the company $15,000 per month in waste and rework costs.
Why Pareto Charts Are Effective
Pareto Charts excel at transforming raw data into actionable insights. Their strength lies in their simplicity and ability to focus attention on the most critical issues, ensuring that resources are used efficiently. In manufacturing, where time and costs are tightly controlled, this prioritization can lead to significant improvements in quality, productivity, and cost savings.
When integrated with other RCA techniques, such as the Ishikawa Fishbone Diagram or 5 Whys, Pareto Charts provide a foundation for deeper analysis and comprehensive problem-solving strategies. For manufacturers aiming to maximize ROI on problem-solving efforts, the Pareto Chart is an invaluable tool.
5 Whys: A Detailed Breakdown
How to Perform the 5 Whys Technique Effectively
The 5 Whys technique is a straightforward yet powerful tool for uncovering the root cause of a problem by repeatedly asking the question "why?" until the fundamental issue is identified. While it is called "5 Whys," the process may require fewer or more iterations depending on the complexity of the problem.
Steps to Perform the 5 Whys Effectively:
- Clearly Define the Problem: Start by articulating the problem in specific, measurable terms. Avoid vague descriptions to ensure the analysis stays focused.
- Example: "The conveyor belt stopped unexpectedly during a production run."
- Ask the First “Why”: Identify the immediate reason why the problem occurred.
- Example: "Why did the conveyor belt stop? Because the motor overheated."
- Continue Asking “Why”: For each answer, ask why that condition occurred. Repeat the process until you identify a root cause that, if resolved, would prevent the issue from recurring.
- Example: "Why did the motor overheat? Because it was running continuously without sufficient cooling."
- Validate the Findings: Ensure that each answer is based on facts, not assumptions. Cross-reference findings with data or observations.
- Example: Maintenance logs confirm that cooling system checks were overdue.
- Develop and Implement Corrective Actions: Once the root cause is identified, propose and execute solutions to eliminate or mitigate the issue.
- Example: Introduce a regular maintenance schedule for cooling systems and install temperature sensors to monitor motor performance.
Real-Life Example: Resolving a Conveyor Belt Stoppage
Scenario: A food packaging facility experiences frequent conveyor belt stoppages, disrupting production and delaying shipments.
- Problem Statement: The conveyor belt stopped unexpectedly, halting production for 30 minutes.
- 5 Whys Analysis:
- Why did the conveyor belt stop?
The motor overheated. - Why did the motor overheat?
The cooling system was not functioning properly. - Why was the cooling system not functioning properly?
The cooling fan was clogged with dust and debris. - Why was the cooling fan clogged with dust and debris?
Preventive maintenance was not performed as scheduled. - Why was preventive maintenance not performed as scheduled?
The maintenance team was understaffed and overwhelmed with emergency repairs.
- Why did the conveyor belt stop?
- Root Cause: Insufficient staffing in the maintenance team led to delays in preventive maintenance, causing the cooling system to fail and the motor to overheat.
- Corrective Actions:
- Hire additional maintenance personnel to handle preventive and emergency tasks.
- Automate maintenance scheduling using a Computerized Maintenance Management System (CMMS).
- Install dust filters on cooling fans and include regular cleaning in the maintenance schedule.
Results: After implementing these solutions, conveyor belt stoppages decreased by 80%, resulting in a monthly production gain of $50,000.
Pitfalls and Best Practices
While the 5 Whys technique is simple and intuitive, its effectiveness depends on careful execution. Below are some common pitfalls and best practices to ensure success:
Pitfalls:
- Stopping Too Early: Ending the analysis after identifying a superficial cause rather than the root cause.
- Example: Blaming the motor's overheating without investigating the cooling system or maintenance practices.
- Lack of Collaboration: Performing the analysis in isolation without involving key stakeholders who have firsthand knowledge of the problem.
- Assuming Instead of Investigating: Jumping to conclusions without verifying the accuracy of answers with data or evidence.
- Ignoring Systemic Issues: Focusing on individual mistakes or equipment failures without addressing broader organizational or systemic problems.
Best Practices:
- Engage a Cross-Functional Team: Involve people from different departments to provide diverse perspectives on the problem.
- Base Conclusions on Data: Use maintenance logs, sensor readings, and production records to validate each answer.
- Focus on Process, Not People: Avoid blaming individuals; instead, look for process-related issues or gaps in training.
- Combine with Other RCA Tools: Use techniques like the Fishbone Diagram or Pareto Chart alongside 5 Whys to ensure thorough analysis.
- Document and Follow Up: Keep detailed records of the analysis and monitor the effectiveness of corrective actions.
Why the 5 Whys Technique Works
The 5 Whys is effective because of its simplicity and focus on direct cause-and-effect relationships. It encourages teams to dig deeper into issues and identify actionable solutions. In manufacturing and industrial automation, where downtime and inefficiencies can be costly, this tool is invaluable for addressing recurring problems and driving continuous improvement. When applied rigorously and combined with data, the 5 Whys can uncover insights that lead to lasting operational enhancements.
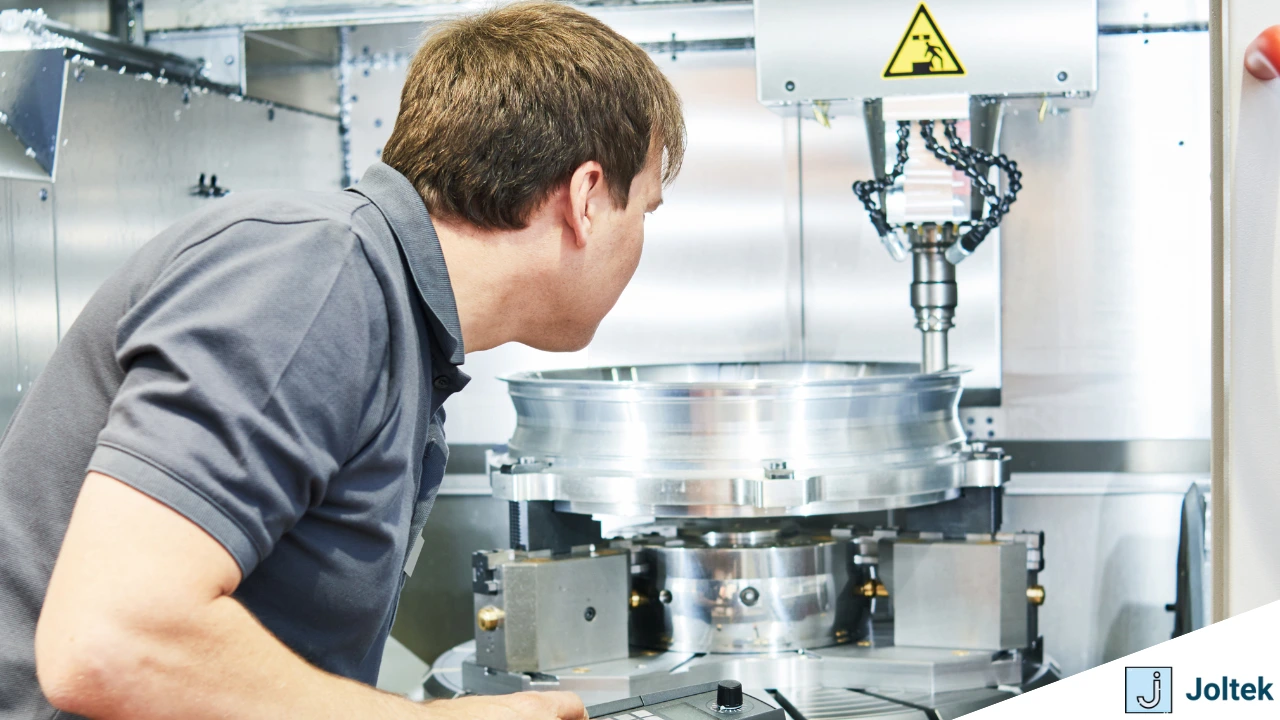
Failure Mode and Effects Analysis (FMEA): A Detailed Breakdown
Definition
Failure Mode and Effects Analysis (FMEA) is a systematic approach for identifying, evaluating, and mitigating potential failure modes in a product, process, or system. The primary goal is to anticipate problems before they occur, assess their potential impact, and prioritize actions to reduce risks. FMEA assigns a Risk Priority Number (RPN) to each failure mode, enabling teams to focus on the most critical issues.
FMEA is widely used in manufacturing and industrial automation for improving reliability, safety, and quality. Its proactive nature makes it a cornerstone in industries where minimizing risk is essential.
Step-by-Step Process
- Define the Scope: Identify the system, product, or process to analyze. Clearly define its purpose, boundaries, and operating conditions.
- Example: A robotic assembly line assembling automotive parts.
- Identify Failure Modes: List potential failure modes for each component or process step. A failure mode describes how something could fail (e.g., "sensor misreads position" or "motor stalls").
- Use historical data, expert input, and brainstorming to ensure comprehensive coverage.
- Determine Effects of Failures: For each failure mode, identify the potential effects on the system or product. Consider how these failures could impact safety, quality, or production.
- Example: A misaligned robot arm could lead to defective assemblies, downtime, or operator safety risks.
- Assign Severity, Occurrence, and Detection Ratings: Evaluate each failure mode based on:
- Severity (S): The impact of the failure on the system or product, rated on a scale (e.g., 1 = negligible, 10 = catastrophic).
- Occurrence (O): The likelihood of the failure happening, rated on a scale (e.g., 1 = unlikely, 10 = very likely).
- Detection (D): The ability to detect the failure before it impacts operations, rated on a scale (e.g., 1 = easily detectable, 10 = undetectable).
- Calculate the Risk Priority Number (RPN): Multiply the ratings: RPN = S × O × D. The higher the RPN, the higher the priority for corrective action.
- Prioritize and Take Action: Focus on high-RPN items. Develop and implement corrective measures to reduce severity, occurrence, or improve detection.
- Example: Introduce predictive maintenance or redesign a component to prevent failure.
- Review and Update: FMEA is a living document. Reassess after implementing changes or when the system or process evolves.
Types of FMEA
- Design FMEA (DFMEA):
- Focus: Potential failures in the design of a product.
- Example: Analyzing the design of a robotic gripper to ensure it securely holds objects under various conditions.
- Process FMEA (PFMEA):
- Focus: Failures in manufacturing or assembly processes.
- Example: Evaluating risks in a robotic assembly line, such as misalignment during welding.
- System FMEA (SFMEA):
- Focus: Failures in integrated systems or subsystems.
- Example: Assessing a robotic assembly line as part of a larger production system, including communication between robots, conveyors, and sensors.
Each type addresses unique aspects of failure, but they often complement each other in complex projects.
Example: Identifying Failure Modes in a Robotic Assembly Line
Scenario: A robotic assembly line in an automotive factory is responsible for assembling door panels. The line occasionally produces defective assemblies, causing delays and rework.
- Define the Scope: Analyze the robotic system responsible for assembling and securing door panels.
- Identify Failure Modes:
- Robot arm misalignment during panel placement.
- Welding equipment failing to secure the panel properly.
- Conveyor system delivering panels with incorrect positioning.
- Determine Effects:
- Misalignment: Defective door panels requiring rework.
- Welding failure: Poor structural integrity, potential safety hazard.
- Conveyor misalignment: Production stoppages.
- Assign Ratings:
- Misalignment: S = 8 (structural defect), O = 6 (frequent), D = 5 (detection depends on visual checks); RPN = 240.
- Welding failure: S = 10 (safety-critical), O = 4 (occasional), D = 7 (detection requires inspection); RPN = 280.
- Conveyor misalignment: S = 7 (minor stoppages), O = 5 (moderate frequency), D = 3 (easily detectable); RPN = 105.
- Prioritize and Take Action:
- High Priority (RPN = 280): Upgrade welding equipment with IoT sensors for real-time monitoring.
- Moderate Priority (RPN = 240): Implement precision alignment tools for robot arms.
- Low Priority (RPN = 105): Schedule regular maintenance for conveyors.
- Review: After implementing solutions, reassess failure modes to confirm the RPNs have been reduced.
Why FMEA is Effective
FMEA’s proactive, quantitative approach makes it a powerful tool for risk mitigation in manufacturing and industrial automation. By focusing resources on the most critical risks, manufacturers can enhance system reliability, prevent costly failures, and ensure safety. Moreover, integrating FMEA with digital transformation tools—such as IoT sensors and predictive analytics—further enhances its effectiveness in modern manufacturing environments.
Fault Tree Analysis (FTA): A Detailed Breakdown
Explanation of FTA and Its Uses in Manufacturing
Fault Tree Analysis (FTA) is a top-down, deductive method for identifying the causes of a specific undesired event, often referred to as the “top event.” It uses a tree-like diagram to visually map the logical relationships between the top event and its potential causes, breaking them down into contributing factors and sub-factors. FTA is particularly effective for complex systems where multiple failures can interact to produce a single outcome.
In manufacturing, FTA is widely used for:
- Systematic Troubleshooting: Identifying the root causes of critical failures in machinery or processes.
- Risk Assessment: Evaluating the probability of system failures and their potential impacts.
- Preventive Action Planning: Designing solutions to eliminate or reduce the likelihood of identified risks.
FTA’s structured approach makes it especially useful in high-stakes environments such as industrial automation, where the failure of a single component can have cascading effects on production.
Example: Troubleshooting a Critical Machine Failure
Scenario: A high-speed bottling machine in a beverage manufacturing facility unexpectedly stops, halting production and risking delivery deadlines.
- Define the Top Event:
- Top Event: Bottling machine stops unexpectedly.
- Construct the Fault Tree:
- Break down the top event into immediate causes using logical "AND" and "OR" gates to represent dependencies and interactions.
- Step 1: Identify high-level causes:
- Power failure (OR gate).
- Mechanical failure (OR gate).
- Sensor failure (OR gate).
- Step 2: Break down each high-level cause into sub-causes:
- Power failure:
- Circuit breaker trip.
- Power supply interruption.
- Mechanical failure:
- Conveyor belt misalignment.
- Motor overheating.
- Sensor failure:
- Faulty proximity sensor.
- Sensor miscalibration.
- Power failure:
- Analyze the Fault Tree:
- Data logs show that the circuit breaker did not trip (rule out power failure).
- Maintenance records indicate that the conveyor belt was recently aligned (unlikely mechanical failure).
- Sensor diagnostic tools reveal irregular readings from the proximity sensor.
- Identify the Root Cause:
- The faulty proximity sensor caused the bottling machine to misinterpret the presence of bottles, triggering an emergency stop.
- Develop and Implement Corrective Actions:
- Replace the faulty sensor and recalibrate the entire sensor system.
- Install a real-time monitoring system to alert operators to future sensor issues.
- Evaluate Results:
- After implementing the corrective actions, machine stoppages decrease by 95%, improving production throughput and reducing downtime costs by $20,000 per month.
Comparison with Other RCA Methods
While FTA is highly effective in specific contexts, its utility differs from other RCA techniques in key ways:
Aspect
Fault Tree Analysis (FTA)
5 Whys
Fishbone Diagram
Approach
Deductive, top-down. Starts with the undesired event and traces causes.
Iterative questioning of “why.”
Categorization of potential causes.
Best Use Case
Complex systems with multiple interacting failure paths.
Simple problems with linear cause-effect.
Problems with many contributing factors.
Visualization
Logical tree structure with AND/OR gates.
No visualization (unless documented).
Visual cause-and-effect diagram.
Level of Detail
High. Maps detailed dependencies and probabilities.
Low to moderate, depending on rigor.
Moderate. Highlights broad categories.
Data Dependency
Requires detailed system knowledge and failure data.
Can be performed with minimal data.
Useful with limited data but benefits from detail.
Key Advantages of FTA:
- Ideal for complex systems where failures result from interdependencies.
- Helps assess probabilities of specific failure paths.
- Provides a structured, visual map of failure scenarios, aiding in communication and planning.
Key Limitations of FTA:
- Time-consuming compared to simpler methods like 5 Whys.
- Requires detailed system data and expertise to construct an accurate tree.
- May not be necessary for straightforward, isolated problems.
Why FTA is Valuable in Manufacturing
Fault Tree Analysis excels in environments where system reliability is critical, and failures involve multiple potential causes. Its logical, visual approach allows manufacturers to systematically evaluate risks, trace complex failure paths, and implement targeted solutions. By combining FTA with other RCA tools—such as Pareto Charts to prioritize issues or FMEA for preventive analysis—organizations can build a comprehensive problem-solving framework that minimizes downtime, improves system reliability, and enhances overall operational efficiency.
Affinity Diagram: A Detailed Breakdown
What is an Affinity Diagram?
An Affinity Diagram is a brainstorming and organizational tool used to group large amounts of data, ideas, or observations into meaningful categories based on their natural relationships. It is especially helpful in situations where information is unstructured or when the root causes of a problem are not immediately clear. By categorizing inputs, the Affinity Diagram helps teams uncover patterns and relationships that may not have been apparent initially.
In manufacturing and industrial automation, Affinity Diagrams are often used during team discussions to address problems such as operational inefficiencies, communication breakdowns, or process bottlenecks. This tool fosters collaboration and is particularly useful for gathering diverse perspectives on complex issues.

Steps to Group Ideas and Identify Root Causes
- Define the Problem or Objective:
- Start with a clear, concise problem statement or goal to focus the brainstorming session.
- Example: "How can we improve communication in our maintenance teams to reduce downtime?"
- Collect Ideas or Data:
- Gather input from team members through brainstorming or surveys. Encourage open-ended contributions without judgment.
- Example: “Shift handovers lack detailed notes,” or “Emergency requests are often delayed.”
- Write Ideas on Cards or Sticky Notes:
- Write each idea or observation on a separate card or sticky note. This allows for flexibility during the categorization process.
- Group Similar Ideas Together:
- Arrange the cards into clusters based on their natural relationships or common themes. Groupings often emerge organically as patterns become evident.
- Example Categories: “Documentation Issues,” “Shift Coordination,” “Tools and Systems.”
- Create Category Labels:
- Assign a label to each group that reflects its overarching theme. Use concise, descriptive names.
- Example: The cluster “Shift handovers lack detailed notes” and “Inconsistent logs” might be labeled as “Documentation Issues.”
- Analyze and Identify Root Causes:
- Review the grouped ideas to identify patterns and deeper issues that contribute to the problem. Use the diagram to brainstorm solutions collaboratively.
- Take Action:
- Develop specific, actionable plans to address the identified root causes based on the insights from the Affinity Diagram.
- Example: Standardizing shift handover logs and implementing digital communication tools.
Example: Streamlining Communication in Maintenance Teams
Scenario: A manufacturing facility is experiencing delays in maintenance tasks due to poor communication among teams, leading to extended downtime and missed production targets.
Steps Using an Affinity Diagram:
- Problem Statement: "How can we improve communication in our maintenance teams to reduce downtime?"
- Brainstormed Inputs:
- “Shift handovers lack detailed notes.”
- “Emergency repair requests are delayed.”
- “Technicians don’t know the exact location of tools.”
- “Supervisors are hard to reach during off-hours.”
- “There’s confusion about which tasks are high-priority.”
- Grouping Ideas:
- Documentation Issues:
- “Shift handovers lack detailed notes.”
- “No standardized process for recording issues.”
- Coordination and Accessibility:
- “Supervisors are hard to reach during off-hours.”
- “Technicians are unclear on priorities.”
- Tools and Logistics:
- “Technicians don’t know the exact location of tools.”
- “Tool tracking system is outdated.”
- Documentation Issues:
- Category Labels:
- Documentation Issues
- Coordination and Accessibility
- Tools and Logistics
- Root Causes Identified:
- Lack of standardized documentation procedures for shift handovers.
- Poor prioritization of tasks during high-demand periods.
- Inefficient tool tracking system.
- Action Plan:
- Documentation: Implement a standardized, digital shift handover log accessible to all team members.
- Coordination: Develop a task prioritization matrix and set up a communication protocol for emergency situations.
- Tools: Upgrade to a real-time tool tracking system integrated with maintenance management software.
Results: Within two months, communication-related delays were reduced by 50%, saving an estimated $10,000 per month in reduced downtime and faster task completions.
Why the Affinity Diagram is Effective
The Affinity Diagram shines in situations where unstructured data or diverse opinions need to be distilled into actionable insights. Its collaborative nature ensures that all team members’ perspectives are considered, fostering ownership and alignment around solutions. By uncovering relationships and patterns, this tool is invaluable for addressing complex problems like communication breakdowns or process inefficiencies in manufacturing. Used alongside other RCA techniques like the Ishikawa Diagram or 5 Whys, the Affinity Diagram adds depth and clarity to problem-solving efforts.
Scatter Diagram: A Detailed Breakdown
How Scatter Diagrams Reveal Correlations Between Variables
A Scatter Diagram, also known as a Scatter Plot, is a graphical representation of two variables plotted on a Cartesian plane to identify possible relationships or correlations between them. Each data point on the chart represents a pair of values, with one variable plotted on the x-axis (independent variable) and the other on the y-axis (dependent variable).
The pattern of the plotted points reveals the nature of the relationship:
- Positive Correlation: As one variable increases, the other also increases (e.g., temperature rise and energy consumption).
- Negative Correlation: As one variable increases, the other decreases (e.g., maintenance frequency and downtime).
- No Correlation: No discernible pattern between the variables.
Scatter Diagrams are particularly useful in manufacturing and industrial automation for uncovering hidden trends and testing hypotheses about relationships between variables. By identifying these correlations, teams can target the root causes of problems with data-backed precision.
Example: Linking Temperature Fluctuations to Equipment Failures
Scenario: A manufacturing plant experiences intermittent equipment failures in its injection molding machines. The maintenance team suspects that temperature fluctuations in the facility might be contributing to the problem.
Steps Using a Scatter Diagram:
- Data Collection:
- Record facility temperature (independent variable) and the frequency of machine failures (dependent variable) over three months.
- Sample data:
- Temperature (°C): 20, 22, 25, 28, 30, 35.
- Failures (per month): 2, 3, 5, 8, 12, 15.
- Plot the Data:
- Plot each pair of values on the Scatter Diagram, with temperature on the x-axis and failures on the y-axis.
- Analyze the Plot:
- The points form a clear upward trend, indicating a positive correlation: as temperature increases, so does the frequency of failures.
- Draw Conclusions:
- The Scatter Diagram confirms that temperature fluctuations are likely contributing to the equipment failures.
- Action Plan:
- Investigate further to understand how temperature affects the equipment (e.g., overheating, material expansion).
- Implement corrective measures, such as upgrading the facility's climate control system or enhancing equipment cooling mechanisms.
Results: After stabilizing the facility's temperature, equipment failures decrease by 70%, improving production efficiency and reducing maintenance costs by $25,000 annually.
Statistical Tools to Complement Scatter Diagrams
While Scatter Diagrams visually highlight correlations, statistical tools can add rigor by quantifying these relationships:
- Correlation Coefficient (r):
- Measures the strength and direction of a linear relationship between two variables.
- Values range from -1 (strong negative correlation) to +1 (strong positive correlation).
- Example: A correlation coefficient of +0.85 between temperature and failures confirms a strong positive relationship.
- Regression Analysis:
- Calculates the equation of the line that best fits the data points on a Scatter Diagram (linear regression).
- Enables predictions based on the relationship between variables.
- Example: Predicting failure rates at temperatures not included in the original dataset.
- Statistical Significance (p-value):
- Determines whether the observed correlation is statistically significant or occurred by chance.
- A p-value below 0.05 indicates a strong likelihood that the relationship is not random.
- Control Charts:
- Monitor variables over time to detect outliers or trends that deviate from expected patterns.
- Useful for tracking whether corrective actions (e.g., temperature stabilization) maintain consistent results.
Why Scatter Diagrams Are Effective
Scatter Diagrams are a simple yet powerful tool for visualizing relationships between variables in manufacturing environments. They help teams identify actionable correlations that might otherwise remain hidden in raw data. By combining Scatter Diagrams with statistical tools like regression analysis and correlation coefficients, manufacturers can transition from observation to prediction, enabling data-driven decisions that improve reliability, efficiency, and cost-effectiveness. In the context of Root Cause Analysis, Scatter Diagrams provide a vital link between evidence and insight, ensuring solutions are both precise and impactful.
Section 4: Supplemental Techniques and Templates
8D Report Template Checklist
Overview of the 8D Problem-Solving Process
The 8D (Eight Disciplines) methodology is a structured problem-solving approach designed to address complex, recurring issues, particularly in manufacturing and industrial automation. It aims to identify root causes, implement corrective actions, and prevent the recurrence of problems. The 8D process is widely used in quality management and aligns well with Root Cause Analysis (RCA) by providing a systematic framework for addressing failures.
The 8D Process:
- D1 – Establish the Team: Assemble a cross-functional team with the necessary expertise and authority to address the problem.
- D2 – Describe the Problem: Clearly define the issue, including its scope, impact, and measurable characteristics.
- D3 – Implement Interim Containment Actions: Introduce temporary fixes to contain the problem and minimize its impact until permanent solutions are in place.
- D4 – Identify Root Causes: Use RCA techniques (e.g., 5 Whys, Fishbone Diagram) to determine the underlying cause of the issue.
- D5 – Develop Permanent Corrective Actions: Propose solutions to address the root cause and prevent recurrence.
- D6 – Implement and Validate Corrective Actions: Execute the solutions and confirm their effectiveness through testing or observation.
- D7 – Prevent Recurrence: Update processes, procedures, or systems to ensure the issue cannot reoccur.
- D8 – Recognize the Team: Acknowledge and document the team’s efforts, ensuring lessons learned are shared across the organization.
How the 8D Process Complements RCA Techniques
The 8D methodology provides structure and accountability, ensuring RCA techniques are applied systematically. For example:
- RCA is central to D4 – Identify Root Causes, where tools like Pareto Charts or FTA are used to analyze problems.
- The 8D approach also emphasizes D7 – Prevent Recurrence, which aligns with the preventive goals of FMEA.
Template Checklist for 8D Reports:
- Problem description (D2): Include data, visuals, and metrics.
- RCA findings (D4): Summarize insights from Fishbone Diagrams or 5 Whys.
- Corrective actions (D5): Document proposed solutions with timelines.
- Validation results (D6): Provide evidence that solutions resolved the issue.
- Process updates (D7): List changes made to prevent recurrence.
DMAIC Template
Explanation of DMAIC (Define, Measure, Analyze, Improve, Control)
DMAIC is a data-driven, iterative process improvement framework rooted in Six Sigma methodologies. It is particularly effective for addressing persistent inefficiencies and quality issues in manufacturing environments. Each phase of DMAIC corresponds to specific steps that drive problem resolution and continuous improvement:
- Define: Clearly define the problem, project goals, and scope. Identify stakeholders and align objectives with business priorities.
- Measure: Collect relevant data to understand the current state and quantify the problem’s impact. Establish baselines for comparison.
- Analyze: Investigate the root causes of the problem using RCA techniques. Analyze data to uncover patterns and relationships.
- Improve: Develop and implement solutions to eliminate root causes. Use data to validate improvements and refine approaches.
- Control: Create controls to sustain improvements over time. Implement monitoring systems and document process changes.
Integration of DMAIC with RCA
DMAIC complements RCA by embedding problem-solving into a broader framework of continuous improvement. RCA techniques are particularly critical in the Analyze phase, where tools like FMEA, Fault Tree Analysis, or Scatter Diagrams can pinpoint root causes. DMAIC’s emphasis on measurement and validation ensures RCA findings translate into actionable, data-backed solutions.
Example of DMAIC in Manufacturing:
Scenario: A manufacturing facility experiences frequent defects in a product assembly line.
- Define: The problem is high defect rates (15%), impacting delivery timelines and customer satisfaction.
- Measure: Analyze defect data to establish baselines. Key metrics: defect rate, downtime, and production output.
- Analyze: Use Pareto Charts to identify that 80% of defects originate from misaligned assembly components. Conduct 5 Whys to reveal that component alignment tools are inconsistently calibrated.
- Improve: Introduce automated calibration systems and retrain operators on tool maintenance. Validate improvements by tracking defect rates over one month.
- Control: Implement weekly calibration checks and update standard operating procedures (SOPs). Set up IoT sensors to monitor alignment tools.
Why DMAIC Enhances RCA
DMAIC’s structured phases ensure that RCA findings are actionable, measurable, and sustainable. By combining RCA’s diagnostic power with DMAIC’s focus on implementation and control, organizations can achieve long-term improvements in efficiency and quality.
Key Takeaways
- 8D Reports provide a step-by-step framework for solving complex problems, ensuring that RCA techniques are used effectively and corrective actions are documented.
- DMAIC ensures that RCA efforts are embedded within a larger process improvement strategy, aligning problem-solving with measurable business goals.
By integrating these supplemental techniques, manufacturers can build a robust, data-driven approach to problem-solving and continuous improvement. Both methodologies emphasize prevention, making them invaluable in achieving operational excellence in manufacturing and industrial automation.
Section 5: RCA Implementation and Best Practices
When to Use RCA: Reactive vs. Proactive
Root Cause Analysis (RCA) can be employed in two distinct scenarios: reactively, to address failures after they occur, and proactively, to prevent problems before they arise. Each approach serves a different purpose and brings unique value to manufacturing and industrial automation.
- Reactive RCA (After Failures):
Reactive RCA is used when an unexpected problem has already occurred, such as equipment breakdowns, production defects, or safety incidents. The goal is to minimize recurrence by identifying and addressing the root causes.- Example: Investigating why a production line stopped unexpectedly due to motor overheating.
- Value: Reduces downtime, prevents repeated failures, and mitigates immediate risks.
- Key Challenge: Time sensitivity, as operations often need to resume quickly.
- Proactive RCA (Preventive Measures):
Proactive RCA aims to identify and eliminate potential issues before they occur. This is often done as part of preventive maintenance, risk assessments, or continuous improvement initiatives.- Example: Using Failure Mode and Effects Analysis (FMEA) to anticipate and mitigate potential failures in a new robotic system.
- Value: Enhances reliability, improves safety, and reduces long-term costs.
- Key Challenge: Requires investment in time, resources, and predictive tools.
Proactive RCA is particularly powerful when integrated with Industry 4.0 technologies, as it allows manufacturers to leverage predictive analytics and real-time data for preemptive decision-making.
The Importance of the Evaluation Step in Problem-Solving
The evaluation step is critical in ensuring that RCA not only identifies root causes but also leads to effective and sustainable solutions. Skipping this step can result in partial fixes or unintended consequences, undermining the effectiveness of the entire RCA process.
- Validating Solutions:
Evaluation ensures that corrective actions address the root cause effectively. For instance, if a conveyor belt’s stoppage was attributed to insufficient lubrication, the evaluation step would confirm whether the newly implemented lubrication schedule eliminates the issue. - Measuring Impact:
Quantitative metrics such as downtime reduction, cost savings, or defect rate improvements are used to assess the success of corrective measures.- Example: After implementing sensor upgrades to prevent motor overheating, maintenance logs and IoT data show a 90% reduction in temperature-related stoppages.
- Identifying Secondary Issues:
The evaluation step can reveal additional, previously hidden problems. For instance, resolving one equipment failure might expose weaknesses in another component or process. - Driving Continuous Improvement:
By analyzing the outcomes of RCA, teams can refine their problem-solving approaches, improve processes, and apply lessons learned across the organization.
Common Pitfalls in RCA
Despite its benefits, RCA can fail to deliver results if certain pitfalls are not avoided:
- Focusing on Symptoms Instead of Root Causes:
- Pitfall: Teams may address surface-level issues (e.g., replacing a faulty component) without exploring the underlying reasons (e.g., why the component failed).
- Solution: Use techniques like the 5 Whys or Fault Tree Analysis to dig deeper into cause-and-effect relationships.
- Inadequate Data Collection:
- Pitfall: Decisions made based on incomplete or inaccurate data lead to ineffective solutions.
- Solution: Ensure thorough data collection from multiple sources, such as maintenance logs, operator feedback, and sensor data.
- Lack of Collaboration:
- Pitfall: RCA performed in silos may overlook critical perspectives or inputs.
- Solution: Engage cross-functional teams, including operators, engineers, and maintenance personnel, to provide diverse insights.
- Rushing the Process:
- Pitfall: Pressure to resume operations can lead to hasty conclusions.
- Solution: Allocate sufficient time to conduct a thorough analysis, even under time constraints.
- Neglecting the Evaluation Step:
- Pitfall: Failing to measure the effectiveness of implemented solutions results in unresolved problems.
- Solution: Include monitoring and follow-up as integral parts of the RCA process.
Tools and Technology to Enhance RCA
Modern manufacturing and industrial automation offer a wide array of tools and technologies that can enhance the effectiveness of RCA:
- IoT Sensors:
- Real-time monitoring of equipment performance (e.g., temperature, vibration, pressure) enables early detection of anomalies.
- Example: IoT sensors identify vibration patterns in a motor that predict imminent failure, prompting proactive maintenance.
- Machine Learning for Predictive Analytics:
- Algorithms analyze historical and real-time data to predict failures and identify patterns that may not be immediately apparent to human operators.
- Example: A machine learning model predicts that frequent conveyor stoppages are linked to humidity levels, prompting installation of dehumidifiers.
- Digital Twin Technology:
- Virtual replicas of physical systems allow teams to simulate scenarios, test hypotheses, and validate corrective actions without disrupting operations.
- Example: Simulating the impact of various maintenance schedules on production efficiency.
- CMMS (Computerized Maintenance Management Systems):
- Centralized platforms for tracking maintenance activities, equipment histories, and RCA findings.
- Example: A CMMS logs recurring issues with a bottling line and automatically schedules preventive maintenance tasks.
- Collaborative Software Tools:
- Tools like Miro, MURAL, or digital whiteboards streamline brainstorming for RCA techniques such as Affinity Diagrams or Fishbone Diagrams, even in remote or hybrid team settings.
Conclusion
Effective RCA implementation requires both a clear understanding of when and how to use it and a commitment to best practices. By addressing failures reactively and proactively, prioritizing evaluation, avoiding common pitfalls, and leveraging modern tools and technology, manufacturers can build robust problem-solving frameworks. This approach not only resolves current issues but also prevents future ones, driving operational excellence and cost efficiency.
Section 6: RCA in Consulting and Systems Integration
How Consulting Services Can Help with RCA Implementation
Root Cause Analysis (RCA) is a powerful tool, but its effectiveness depends on disciplined implementation, deep expertise, and alignment with broader organizational goals. Consulting services play a critical role in helping manufacturing facilities achieve these outcomes by offering tailored solutions, external expertise, and structured methodologies.
- Identifying Gaps and Opportunities:
Consultants assess existing processes and identify gaps in how RCA is currently applied. For example, a facility may struggle with recurring failures due to inconsistent use of tools like the 5 Whys or Fishbone Diagrams. Consultants can pinpoint these issues and recommend corrective actions. - Developing Customized RCA Frameworks:
Every facility has unique challenges. Consultants design RCA frameworks that align with the organization’s specific equipment, workflows, and culture.- Example: In a food manufacturing plant, a consultant might integrate RCA into safety compliance protocols to address contamination risks.
- Facilitating RCA Sessions:
External facilitators bring objectivity to RCA sessions, reducing bias and ensuring a thorough analysis. Their experience across industries also provides insights into best practices and innovative solutions. - Embedding RCA into Broader Strategies:
Consultants help integrate RCA into lean manufacturing, Six Sigma, or Industry 4.0 initiatives, ensuring it becomes a cornerstone of operational excellence rather than a reactive measure.
Upskilling Teams in RCA Techniques: Training Programs and Workshops
One of the most impactful ways consultants add value is by empowering internal teams with the skills and knowledge to perform RCA independently. This is achieved through training programs and workshops tailored to the organization’s needs.
- Fundamentals of RCA:
Training programs introduce the principles and methodologies of RCA, ensuring all participants understand the importance of identifying root causes rather than addressing symptoms. - Hands-On Workshops:
Practical sessions guide teams through real-world scenarios, demonstrating how to use tools like the 5 Whys, Pareto Charts, or Fault Tree Analysis in their specific operational contexts. - Certification Programs:
Offering certifications in RCA or related methodologies like FMEA or Six Sigma enhances employee credentials and builds organizational capability. - Leadership Training:
Upskilling supervisors and managers ensures RCA becomes ingrained in the culture. Leaders are trained to facilitate RCA sessions, encourage cross-functional collaboration, and drive continuous improvement initiatives. - On-the-Job Support:
Post-training, consultants provide on-the-job coaching to reinforce learnings and address challenges as teams begin applying RCA in real situations.
Integrating RCA into Manufacturing Systems
For RCA to deliver sustained value, it must be embedded within the organization’s technological and operational ecosystems. This integration ensures that RCA findings are actionable, measurable, and continuously accessible.
- MES and SCADA Integration:
- Manufacturing Execution Systems (MES) and Supervisory Control and Data Acquisition (SCADA) systems collect vast amounts of real-time production data. By integrating RCA with these systems, manufacturers can identify root causes faster and implement corrective actions more effectively.
- Example: An MES captures data on machine performance and defects. RCA tools integrated with MES data pinpoint that high defect rates in a bottling line are caused by irregular sensor calibrations.
- Automated Alerts and RCA Triggers:
- SCADA systems can generate automated alerts when performance thresholds are breached, such as unusual vibration or temperature levels. These alerts serve as immediate triggers for RCA investigations.
- Example: A SCADA system detects a spike in motor temperatures and automatically logs the event in the MES, prompting an RCA session to determine the root cause.
- IoT and Predictive Analytics:
- Internet of Things (IoT) sensors provide continuous monitoring of equipment and processes, enabling predictive analytics to forecast potential failures. RCA frameworks use this data to address root causes proactively.
- Example: IoT sensors on a packaging machine identify wear patterns in components, leading to preemptive maintenance scheduling based on RCA findings.
Data-Driven Insights for Continuous Improvement
Integrating RCA with data-driven tools transforms it into a dynamic process that not only solves problems but also fosters continuous improvement.
- Centralized Data Platforms:
- Platforms like ERP (Enterprise Resource Planning) or CMMS (Computerized Maintenance Management Systems) centralize RCA-related data, ensuring teams have access to historical findings and trends.
- Example: A CMMS highlights recurring failures in a specific machine, enabling engineers to perform a meta-RCA to address systemic issues.
- Analytics Dashboards:
- Dashboards display RCA outcomes, such as resolved issues, recurring problems, and the impact of corrective actions. These insights help track progress and identify areas for further optimization.
- Example: A dashboard shows a 40% reduction in downtime after implementing RCA findings, justifying further investment in advanced diagnostic tools.
- Feedback Loops:
- RCA findings feed back into preventive measures, design improvements, and training programs, creating a self-reinforcing loop of continuous improvement.
- Example: After identifying poor operator training as the root cause of a defect, the training program is updated, reducing similar issues in other areas.
Why RCA Consulting and Integration Matter
Consulting and systems integration services amplify the power of RCA by aligning it with organizational goals, upskilling teams, and embedding it into technological ecosystems. This holistic approach ensures RCA is not just a problem-solving tool but a strategic enabler of operational excellence, cost efficiency, and innovation in manufacturing. By leveraging expert guidance and modern technologies, organizations can turn RCA into a competitive advantage.
Section 7: FAQs About RCA
Can You Always Identify the Root Cause?
No, it is not always possible to identify the root cause of a problem completely. Complex systems, data limitations, and human factors can make certain root causes elusive. However, the goal of Root Cause Analysis (RCA) is to identify actionable causes that significantly reduce the likelihood of recurrence, even if absolute certainty isn’t achievable.
- Challenges:
- Interconnected systems with multiple contributing factors.
- Insufficient or incomplete data.
- Difficulty in replicating failure conditions.
- Best Practices:
- Use multiple RCA tools to explore potential causes from different angles (e.g., Fishbone Diagram, Fault Tree Analysis).
- Collect comprehensive data through sensors, logs, and operator feedback.
- Focus on addressing the most likely or impactful causes even if some ambiguity remains.
What Is the Best Tool for RCA in Manufacturing?
The "best" tool for RCA in manufacturing depends on the problem’s complexity, the data available, and the context. Different tools excel in different scenarios:
- Fishbone Diagram (Ishikawa): Best for brainstorming and categorizing potential causes in a structured manner.
- Use Case: Investigating recurring defects in an assembly line.
- Pareto Chart: Ideal for prioritizing issues based on their frequency or impact using the 80/20 rule.
- Use Case: Identifying which 20% of failures cause 80% of production downtime.
- 5 Whys: Simple and quick for linear cause-and-effect problems.
- Use Case: Diagnosing why a conveyor belt stopped unexpectedly.
- Failure Mode and Effects Analysis (FMEA): Proactive tool for analyzing potential failure modes in a system or process.
- Use Case: Designing a new automated line to minimize risks before deployment.
- Fault Tree Analysis (FTA): Best for mapping out complex failure scenarios with interdependent causes.
- Use Case: Troubleshooting intermittent power losses in a robotic system.
Selecting the best tool often involves combining multiple techniques to ensure thorough analysis.
How Do You Isolate the Root Cause of a Malfunction?
Isolating the root cause of a malfunction requires a systematic approach that combines data, expertise, and problem-solving tools. Here’s a step-by-step guide:
- Define the Problem:
- Clearly articulate the malfunction, including its scope, impact, and observable symptoms.
- Example: “A robotic arm in the assembly line stalls intermittently.”
- Gather Data:
- Collect relevant data from sensors, logs, maintenance records, and operator feedback.
- Example: IoT sensors show that stalling occurs when the arm rotates beyond a certain angle.
- Use RCA Techniques:
- Apply tools like the 5 Whys, Fishbone Diagram, or FTA to analyze potential causes systematically.
- Example: “Why does the robotic arm stall? Because the motor overheats. Why does the motor overheat? Because of insufficient lubrication.”
- Test Hypotheses:
- Validate potential root causes by testing or simulating conditions.
- Example: Reapply lubrication and monitor the motor’s temperature during operation.
- Eliminate Confounding Factors:
- Rule out secondary issues or unrelated symptoms that could mask the true root cause.
- Example: Check whether sensor miscalibration contributes to false error signals.
- Confirm the Root Cause:
- Use data and observation to verify the solution’s effectiveness.
- Example: After applying corrective measures, the robotic arm operates without stalling for two weeks.
Examples of Successful RCA in Manufacturing
- Reducing Defects in a Beverage Bottling Line:
- Problem: High defect rates in sealed bottles.
- RCA Tool: Fishbone Diagram and Pareto Chart.
- Root Cause: Misaligned sealing machines and inconsistent bottle heights.
- Solution: Recalibrated machines and implemented stricter material quality checks.
- Result: Defects reduced by 60%, saving $50,000 annually.
- Eliminating Downtime in an Automotive Assembly Plant:
- Problem: Repeated conveyor belt stoppages.
- RCA Tool: 5 Whys and Fault Tree Analysis.
- Root Cause: Overloaded circuits due to outdated wiring.
- Solution: Upgraded wiring and installed load-balancing systems.
- Result: Downtime decreased by 90%, improving production efficiency.
- Improving Safety in a Food Processing Facility:
- Problem: Frequent employee slips in a packaging area.
- RCA Tool: Affinity Diagram.
- Root Cause: Condensation from nearby cooling units creating slippery floors.
- Solution: Installed better drainage and anti-slip mats.
- Result: Incident rates dropped to near zero, reducing liability costs.
- Enhancing Reliability of Injection Molding Machines:
- Problem: Frequent part rejections due to improper dimensions.
- RCA Tool: Scatter Diagram and FMEA.
- Root Cause: Inconsistent cooling times caused by fluctuating water pressure in the cooling system.
- Solution: Installed pressure regulators and automated monitoring.
- Result: Rejection rates dropped by 75%, saving $100,000 in rework annually.
Conclusion
RCA is a vital tool for solving problems in manufacturing, but its effectiveness depends on understanding its nuances and applying the right tools for the job. While identifying the root cause is not always straightforward, disciplined approaches, data-driven techniques, and advanced technologies can significantly improve outcomes. By mastering RCA and learning from successful implementations, manufacturers can achieve greater reliability, efficiency, and cost savings.
Section 8: Conclusion
Recap of the Importance of RCA
Root Cause Analysis (RCA) is more than just a problem-solving tool; it is a cornerstone of operational excellence in manufacturing and industrial automation. By systematically identifying and addressing the underlying causes of issues, RCA enables organizations to reduce downtime, improve quality, enhance safety, and optimize costs. Whether applied reactively to resolve failures or proactively to prevent them, RCA drives sustainable improvements and strengthens the resilience of manufacturing systems.
Throughout this guide, we’ve explored various RCA techniques, from the Ishikawa Fishbone Diagram to Scatter Diagrams, and supplemental frameworks like 8D Reports and DMAIC. These tools, when integrated with modern technologies such as IoT sensors and machine learning, offer manufacturers powerful solutions to tackle complex challenges and foster continuous improvement.
Implementing RCA effectively requires more than tools and processes—it demands expertise, collaboration, and a commitment to long-term success. Whether you’re addressing recurring defects, optimizing maintenance, or integrating RCA into digital transformation initiatives, the value of RCA lies in its ability to deliver actionable insights and lasting results.
Call to Action: Consulting Services, Training, and Systems Integration Support
At Joltek, we specialize in helping manufacturers unlock the full potential of Root Cause Analysis through tailored consulting, comprehensive training programs, and seamless systems integration. Our services include:
- Consulting: We assess your operations, identify gaps, and implement customized RCA frameworks to address your unique challenges.
- Training: Equip your teams with the skills to perform RCA effectively through hands-on workshops, certifications, and on-the-job coaching.
- Systems Integration: Embed RCA into your manufacturing systems with MES/SCADA integration, IoT-enabled analytics, and predictive maintenance solutions.
By partnering with us, you gain access to industry expertise and cutting-edge technologies that ensure RCA is not just a tool but a strategic enabler of your operational goals. Let us help you transform your approach to problem-solving and drive measurable improvements in efficiency, quality, and profitability.
Contact us today to schedule a consultation or learn more about our services.
Links to Resources and Further Reading
Expand your knowledge of RCA and related methodologies with these valuable resources:
- Fishbone Diagrams in Manufacturing: A comprehensive guide to using Ishikawa Diagrams for RCA.
- Pareto Analysis for Manufacturing: Prioritizing issues to maximize impact.
- Failure Mode and Effects Analysis (FMEA): Proactive risk management for systems and processes.
- Integrating RCA with Industry 4.0: How IoT and predictive analytics enhance RCA effectiveness.
- 8D Problem-Solving Templates: Free resources for structured problem resolution.
For more insights, tools, and real-world case studies, visit [Joltek’s Knowledge Hub] (insert link).
Root Cause Analysis is a journey, and with the right strategies and support, it can become a transformative force for your organization. Let’s make operational excellence your new standard!
References
Below is a compiled list of references to support the content on Root Cause Analysis (RCA), including books, case studies, industry reports, and authoritative websites. These resources provide further reading and validation for the methodologies, tools, and examples discussed.
Books
- "Root Cause Analysis: Improving Performance for Bottom-Line Results" by Robert J. Latino, Kenneth C. Latino, and Mark A. Latino
- A comprehensive guide to RCA techniques and their application in industrial settings.
- "The Lean Six Sigma Pocket Toolbook" by Michael L. George, John Maxey, David Rowlands, and Mark Price
- A practical reference for tools like DMAIC, FMEA, and Pareto Charts.
- "Quality Toolbox" by Nancy R. Tague
- Covers a wide range of quality tools, including the Fishbone Diagram and Scatter Plots.
- "The Goal: A Process of Ongoing Improvement" by Eliyahu M. Goldratt and Jeff Cox
- Offers insights into operational excellence and problem-solving approaches.
Case Studies
- "Reducing Downtime in Manufacturing: An RCA Case Study" (XYZ Industry Publication)
- Details how RCA and IoT sensors reduced unplanned stoppages by 50% in an automotive plant.
- "Implementing FMEA for Risk Mitigation in Food Processing" (Case Study by Food Safety Tech)
- Highlights the role of FMEA in proactively identifying risks in a high-speed production line.
- "Using 8D Problem-Solving to Improve Quality in Assembly Operations" (Case Study by Quality Progress Magazine)
- A real-world example of structured problem-solving reducing defect rates by 40%.
Industry Reports
- "The State of Industry 4.0: How IoT and Analytics Are Driving Predictive Maintenance" by Deloitte Insights
- Explores the role of predictive analytics and IoT in enhancing RCA.
- "2024 Manufacturing Trends Report" by McKinsey & Company
- Covers the importance of RCA and related methodologies in addressing downtime and quality issues.
- "Cost of Downtime in Manufacturing" by Aberdeen Group
- Quantifies the financial impact of unplanned downtime and highlights RCA as a key mitigation strategy.
Authoritative Websites
- American Society for Quality (ASQ) - www.asq.org
- A reliable source for articles, tools, and case studies on RCA techniques like FMEA, Fishbone Diagrams, and more.
- Six Sigma Online - www.sixsigmaonline.org
- Offers free resources and templates for RCA methodologies like DMAIC.
- Root Cause Analysis Guidance by NASA - www.nasa.gov
- Insights into RCA applications in engineering and aerospace industries.
- National Institute of Standards and Technology (NIST) - www.nist.gov
- Resources on quality management and RCA in advanced manufacturing systems.
- Global IoT Analytics - www.iot-analytics.com
- Articles and reports on how IoT enhances RCA in manufacturing.
Templates and Tools
- Fishbone Diagram Templates (Lucidchart) - www.lucidchart.com
- Ready-to-use templates for creating Fishbone Diagrams.
- 8D Report Templates (ASQ) - www.asq.org/quality-tools/8d-report
- Structured templates for 8D problem-solving documentation.
- FMEA Excel Templates (Quality-One) - www.quality-one.com/fmea-template/
- Downloadable resources for conducting Failure Mode and Effects Analysis.
For detailed access to these resources, consider exploring their respective publications and websites. These references provide the foundation for building a robust understanding of RCA techniques and their practical applications in manufacturing and industrial automation.